North Sea operators have a convergence of interests to find alternative power sources for their offshore platforms and subsea systems. Gas turbines typically power offshore oil and gas platforms and drilling rigs by generating electricity on site. Turbine emissions, however, counter global emission reduction goals, and emissions from fuel transport vessels exacerbate the problem.
Alternative offsite power sources eliminate platform emissions, increase rig space, reduce platform weight, and improve its environment. Offshore wind farms have been adopted as direct power sources for offshore production, and wave energy is being investigated for locally powering remote subsea systems.
Offshore wind power
Two cases of a theoretical study of fuel consumption simulations in the Southern North Sea on the UK continental shelf (SNS-UKCS) compared gas turbine power generation with offshore wind power using published data.1 In Case 1, a 100-Mw offshore wind farm connected to five oil platforms reduced overall fuel consumption by 21-32% over 1 year (see Table).
Case 2 assessed technical integration of gas turbines and wind power. The study assumed that turbine power would still be required under all wind conditions and sought to optimize power distribution for maximum efficiency and emissions reduction. In addition to emission reductions from the wind turbines, running a second gas turbine intermittently (start-stop) to fill in power demand reduced emissions further by limiting operation to its most efficient range. A generator running above its optimum load range wastes power, whereas a generator running below optimum load causes wet stack, where lower engine temperatures fail to efficiently burn fuel. Using the second turbine in start-stop operation to keep both main and second turbines in peak operational mode, gas efficiency rose from 25.6% to 30.1%.
Peak efficiency came by cycling the second turbine on and off 543 times during the year, increasing wear and maintenance costs (which were not captured in the analysis). Also not addressed was the additional cost of this unit compared with costs (in gas consumption and additional emissions) by using only one unit and running it inefficiently. Other outstanding issues to address for combined power distribution were a sharp drop-off of fuel savings with reduced wind power, unreliable supply of constant wind power, and reduction of gas turbine efficiency under low load.
Overall, the study showed that adding wind power to offshore fields can effectively reduce emissions, but that further study is required to efficiently merge them into a dual-power scheme. The problems are particularly acute when trying to incorporate wind energy into aging platforms which do not have upgraded operational systems to respond to fluctuating wind supply.
Wind-powered North Sea fields
Equinor reports that oil and gas installations on the Norwegian continental shelf (NCS) emit about 13 million tonnes/year (tpy) of CO2 equivalent from flaring and gas-turbine power generation. Combined, they account for nearly a quarter of Norway’s total greenhouse gas emissions.
Operators on the Norwegian continental shelf pay tax on emissions which can reach about $76/tonne. Fields close enough to shore, such as Troll, Gjøa, Martin Linge, and Johan Sverdrup, reduce emissions by obtaining electricity from onshore generators (Fig. 1). Johan Sverdrup, for example, emits 0.67 kg/bbl of CO2 using power from shore, compared with an average of some 9 kg/bbl on the Norwegian continental shelf and 15 kg/bbl globally for offshore infrastructure powered by platform-mounted gas turbines. Martin Linge is the furthest field electrified from shore, using a 163 km subsea cable.
Equinor-operated Gullfaks and Snorre are similarly distant from shore as Martin Linge but will be powered by the Hywind Tampen wind park about 140 km off the Norwegian coast in 260-300 m of water (Fig. 2). It is the world’s first wind farm to power offshore oil and gas platforms. Installed on floating concrete structures with a shared anchoring system, the farm has 11 wind turbines with a total 88-Mw generating capacity. The wind turbines are connected in a 2.5 km-long network with 66-kv capacity. Equinor now operates 47% of the world’s floating wind capacity.
Hywind Tampen is estimated to meet about 35% of the annual electricity power demand of Snorre A and B platforms, and Gullfaks A, B, and C platforms. This percentage should increase during periods of high wind, and the wind farm is expected to offset 200,000 tpy of CO2 emissions and 1,000 tpy of NOx emissions by displacing gas turbine power.
Neptune Energy Norge AS is also developing offshore wind power for its platforms. On Dec. 12, 2022, Neptune reported that it signed an MOU with Ørsted AS and Goal7 Ltd. to explore powering future Neptune-operated UK North Sea hubs with wind. The integrated energy hubs can also potentially power existing oil and gas production assets, carbon storage, and hydrogen production.
Ørsted’s Hornsea 1 and 2 offshore wind farms are in the largest offshore wind zone in the world, about 120 km and 89 km off Yorkshire, England, in the North Sea, respectively (Fig. 3). Hornsea 1 produces 1.2 Gw through 174 wind turbines and entered operation in 2019. Hornsea 2, the world’s largest windfarm, produces 1.3 Gw through 165 wind turbines and entered full operation in August 2022.
Wave energy
A wave energy converter (WEC) is a mechanical device which captures wave energy and converts it to power through a vernier hybrid machine direct-drive generator (see box). A WEC developed by Mocean Energy Ltd. through funding by Wave Energy Scotland (WES), a government-led initiative started in 2014 to drive technical innovation in the sector, produces 10 kw of power through a floating structure consisting of two hulls joined by a hinge.2-3 Wave forces on the hull cause a relative motion around the hinge that drives the generator. The WEC provides renewable power for subsea applications such as control systems, remotely operated vehicles, and autonomous underwater vehicles (AUV).
Detailed hydrodynamic studies optimized the devices asymmetric shape and sloping forward and aft sections to assure greatest wave power absorption under a broad range of wave types (Fig. 4). The WEC is most efficient in small-to-medium waves, but its sloping end design provides survivability in extreme waves.
During high waves, the WEC dives and ducks under the brunt force of the wave. This feature reduces mooring loads and, using the relative motion of the WEC against itself (rather than the mooring line) for power generation, relaxes mooring requirements, making it suitable for deepwater-compliant mooring systems. Extreme waves are handled by disengaging the hinge to allow the hulls to rotate independently without causing end-stop impacts against the generator.
The Blue Star 10 WEC prototype underwent 5 months of sea trials in 2021 at the European Marine Energy Center Ltd. (EMEC), in the Orkney Islands, Scotland. It is 19.3 m long, 7.6 m high, 4.6 m wide, and weighs 38 tonnes (Fig. 5). It produces 10 kw nominal power, stores 33 kw-hr of energy, and communicates through 3G, 4G, VHF, and wi-fi. The prototype generated power and provided data on machine performance and operation over the summer months.
During the trials, Blue Star 10 demonstrated survivability in extreme waves with the hinge successfully disengaging to protect the power generator. The mean AC electrical power produced by the WEC under differing wave conditions matched modeled power predictions. Although Blue Star 10 is rated for 10 kw, only 4 kw was generated during 1.5 m waves, the maximum encountered wave height reported in the test.
In 2020, a UK industry collaborative project, Renewables for Subsea Power (RSP) brought together three technology developers (Mocean Energy, Verlume Ltd., and Transmark Subsea AS), a service company (Baker Hughes), and two operators (Harbour Energy PLC, formerly Chrysaor, and Serica Energy PLC) to develop a fully integrated standalone subsea system to provide local power and communications for subsea infrastructure, replacing long umbilical cables that connect subsea assets to power and communication hubs.
The system contains subsea energy storage (HALO), power delivery to subsea production control systems, and AUV for monitoring and maintenance, all using WEC power (Fig. 6). The project is being developed in three phases: Phase 1 was for front-end engineering design (FEED), Phase 2 is for the build and onshore commissioning of subsystems, and Phase 3 is for full-system deployment and trials. Phase 1 completed in 2021, Phase 2 completed in third-quarter 2022, and Phase 3 is ongoing and scheduled to complete in second-quarter 2023.
Over-the-horizon communication is primarily with wi-fi/3G/4G (depending on location) or radio back up. HALO also has a backup through-water acoustic system in case the WEC becomes immobile, or it loses communication with the WEC.
EuropeWave, a research and development program for wave energy technology that, like WES, implements precommercial procurement programs, plans to field test Blue Horizon 250, a 250-kw nominal power rated WEC. It is currently in FEED for a prototype device. A 12-month open-water demonstration at the Biscay Marine Energy Platform off the coast at Armintza, Spain, and at EMEC is scheduled to start in 2023.
References
- Anyanga, S.P., “Design of an Integrated Sustainable Energy System,” ADIPEC, Abu Dhabi, UAE, Oct. 31-Nov. 3, 2022.
- Crossland, I.R., Slorach, P., Caio, A., Parle, J., McNatt, C., “Innovating Industry Lead Project for Renewables for Subsea Power,” SPE-210910-MS, ADIPEC, Abu Dhabi, UAE, Oct. 31-Nov. 3, 2022.
- McNatt, J.C. and Retzler, C.H., “The performance of the Mocean M100 wave energy converter described through numerical and physical modelling,” International Marine Energy Journal, Vol. 3, No. 1, May 14, 2020.
- Arish, N. and Teymoori,, V., “Development of Linear Vernier Hybrid Permanent Magnet Machine for Wave Energy Converter,” IJE Transactions B: Applications, Vol. 33, No. 5, May 2020, pp. 805-813.
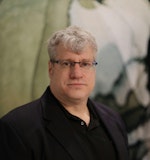
Alex Procyk | Upstream Editor
Alex Procyk is Upstream Editor at Oil & Gas Journal. He has also served as a principal technical professional at Halliburton and as a completion engineer at ConocoPhillips. He holds a BS in chemistry (1987) from Kent State University and a PhD in chemistry (1992) from Carnegie Mellon University. He is a member of the Society of Petroleum Engineers (SPE).