Simultaneous fracturing (simulfracs) treats multiple wells simultaneously, splitting operations among wells to minimize downtime in stimulation operations. Two recent studies compared simulfrac operational efficiencies to zipper fractured completions (zipperfracs) in the Permian and Bakken basins. The results showed that in either region, simulfracs were faster (typically 30%) and cheaper (typically 3-6%), but only when treating rates per well were comparable.
A study of microseimic responses of simulfracs versus zipperfracs suggests that stimulation effectiveness from simultaneous fracturing treatments may be lower, with smaller observed stimulated reservoir volume (SRV) in the simulfracs based on microseismic imaging. This may be due to the increased efficiency of the simulfracs, with the lower microseismicity related to shorter leak-off time between stimulations.
Simulfracs
With economic realities pushing hydraulically fractured completions towards ever higher efficiency and lower costs, multiple pad operations are being combined and optimized to save time and material costs. Zipperfracs were the first step-change in time savings; splitting stimulation operations between two wells, but with pumping restricted to one well at a time. Operators sought to further reduce completion time by increasing treating rate. Higher treating rates, however, led to increased friction pressures, resulting in additional spend on hydraulic horsepower, friction reducers, and high-pressure (15,000 psi) control equipment.
Simulfracs save time by combining fracture operations rather than increasing pump rate. The most basic example of this includes two frac crews working simultaneously on the same pad, treating two wells at once with fluid split between the wells. Due to the additional cost of the second crew and duplication of equipment, this approach does not achieve much cost savings. It is essentially just two frac crews flying in tight formation.
A more efficient use of the simulfrac technique employs one crew who splits duties while fracturing two wells. Additional equipment may still be required depending upon the number of wells simultaneously stimulated. Fig. 1 shows general rig-up and procedures for zipperfrac vs. simulfrac operations for four wells in a typical plug and perforation (PnP) operation. The simulfrac ideally requires additional sand silos, blenders, frac pumps, wireline units, and cranes (though some equipment can be doubled-up with loss of efficiency), but the flow chart of operations shows less down time as part of the simulfrac operation. By splitting flow among the wells, PnP operations are performed simultaneously with stimulation treatments throughout the job.
Two recently published studies cover simulfrac operations. Chevron Corp. performed simulfracs in the Permian basin and compared results with zipperfracs in the same area.1 QEP Resources Inc. and Halliburton Co. performed simulfracs in the Bakken with sliding sleeve technology and in the Permian with PnP technology.2 As with the Chevron study, simulfracs efficiency was compared with zipperfracs on the same pad.
Chevron Permian simulfracs
Chevron trialed simulfracs on a six-well pad in an established area with significant benchmark data. All wells were in the same formation. The pads had even numbers of wells, allowing for an even split between dedicated pump banks and zipper manifolds. An additional crane, wireline unit, and pump down kit were added to the pad for simulfrac operations. No additional pumps were used for the simulfracs due to existing pad size limitations and cost of expanding pads.
A single crew performed simulfracs with a single blender. Flow meters were installed on each pump bank and the banks were isolated to treat wells at different pressures. Each well had its own treatment plan, but the proppant type and concentration were similar due to the limitations of a single blender.
Safety concerns included operating only one crane at a time, spacing cranes out to prevent boom collisions, using dedicated zipper frac manifolds to prevent accidentally lining up both pump banks to one well, and operating only one well’s hydraulic valve at a time to ensure the correct well is lined up at any given time.
The zipper frac treatment design was 8,600 psi @ 90 bpm. Simulfracs were limited to 8,500 psi @ 60 bpm per well due to the pump bank split between wells in the simulfracs. The cluster design was subsequently downsized to keep rate per perforation cluster constant between zipper frac and simulfrac treatment designs. This shortened the stage length and increased the number of stages per well for simulfracs, increasing overall completion time per well for the simulfracs.
Table 1 shows results of the first trial pad. Simultaneous operations completed more lateral length per day even with the higher number of stages per well. Cycle time was reduced 29%. The table also shows that simulfracs require less friction reducer due to lower treating rates, contributing to 3% total material cost savings per pad.
Taking advantage of reduced friction pressure at lower treating rates, simulfrac treatment rate was bumped up to 70 bpm per well, and the simulfrac achieved a maximum total 2,959 ft lateral length per day, about 700 ft longer than the maximum zipperfrac operation.
Simulfracs proved out on all stage types for the first six trial pads using different stimulation designs, fluid intensity, and treating pressures. Single-well zipperfracs were only used on pads with odd numbers of wells. Simulfrac resulted in an average 30% increase in lateral ft/day with an average cost reduction of 6% per pad. Operations and the water supply chain consistently handled 140 bpm treating rates. The business unit leveraged simulfracs to deliver six additional wells in the fiscal year.
Based on these positive results, simulfracs were trialed in other areas of the Permian basin. Some of the highest reservoir pressure and most difficult formations in Chevron’s portfolio were treated, and treating pressures were successfully reduced such that surface equipment pressure-control rating requirements were reduced in some areas from 15,000 psi to 10,000 psi. The success of these trials convinced Chevron to pursue full implementation of simulfracs.
QEP Bakken simulfracs
QEP Resources completed its first simulfrac operation in North Dakota’s Bakken oil field. The wells were uncemented with sliding sleeves. High pressure drops through sliding sleeves require relatively low treating rates compared with PnP completions, and simultaneous operations leverage completion time efficiency when rates are limited.
The trial compared simulfrac wells (Foreman 1 and Foreman 2) with zipperfrac wells (Foreman 3 and Foreman 4). Operations used one hydraulic fracturing fleet with minimal changes to equipment. Rates were limited to 35 bpm for all wells with 200-ft stage lengths.
Simulfrac wells were more efficient, pumping on average 80% more slurry per day (Table 2). Completion days were reduced from 5 to 3. The simulfrac wells also pumped more slurry volume per day than all other wells completed by QEP over the course of a year in the area.
QEP Permian simulfracs
QEP used the PnP technique in the Permian basin, resulting in higher rates and volumes than completions in the Bakken. Rate limits were based on surface equipment ratings and available hydraulic horsepower. Zipperfracs in the area approached 100 bpm/well, but this rate is not practically achievable per well for simulfracs using conventional pumping techniques. A split-fluid technique, therefore, was employed to increase simulfrac treating rates to approach those of the zipperfracs.
Split-fluid pumping splits flow between a concentrated slurry and clean fluid. Discharge rates from the blender are supplemented with additional clean fluid, increasing total downhole rate. A basic split-fluid scheme is shown in Fig. 2.3 For clarity, the illustration shows pumps taking slurry and clean fluid from separate blenders and discharging to the high-pressure manifold, which subsequently discharges to the zipper manifold. In the QEP operation, a more complicated arrangement was used in which each well received one dirty and one clean line from their respective bank of pumps. Flow was monitored at each well, and adjustments could be made in real time altering slurry concentration at the well. This arrangement allowed for a rapid response to changes in each well’s rate and proppant and provided the ability to pump different treatments to each well.
One van supervisor and pump operator controlled operations through updated software which monitored fluid and sand for multiple wells, controlled pumps for different wells, and monitored multiple treatment charts. Two wireline crews were on location. As with the Chevron completions, sand and water logistics were planned to maintain supply during the simultaneous operations.
Table 3 shows results from pads completed with zipperfracs and simulfracs. Like the Chevron study, the simulfracs generally pumped more slurry per day and had longer lateral lengths completed per day.
Comparing multiple fracturing operations allowed analysis of the economic benefits of simulfracs. Pad operations were compared between 90-bpm zipper wells, 160 bpm split-fluid simulfrac wells, and 100-bpm simulfrac wells. Fig. 3 shows cost efficiency ($/ft) among the completions factoring frac crew rental, chemical, water costs, proppant, wireline, and ancillary costs. Stimulation design was 27 bbl/ft with 1,600-lb/ft proppant loading. Cost comparisons were made using standardized completion operation costs rather than actual well costs to eliminate local operation variations not associated with completion type. Pad operations were standardized to eight-well pads with 2-mile lateral lengths while using actual stage lengths.
The 100 bpm simulfrac completion was the least cost efficient, but the 160 bpm simulfrac was the most efficient, showing that the advantages of simultaneous operations are not enough to offset higher-rate zipperfracs. At comparable rates, however, simulfracs were significantly more efficient. Completion time for the 180 bpm simulfrac wells was 19 days, compared with 34 days for the 90 bpm zipperfrac.
Simulfrac stimulation effectiveness
Effects of simulfracture stimulations on fracture geometry relative to other techniques are still unclear. Simultaneously stimulating adjacent wells could affect stress states differently than would be expected with conventional single or zipper fracture treatments, possibly altering fracture geometries. Quantifying these effects could aid in identifying ideal well pairs for simultaneous stimulation.
Vermylen et. al. performed a microseismic and stress evolution study comparing stimulation techniques in a Barnett shale pilot.4 Seismic events during slickwater fracturing treatments were compared between conventional single-well fracturing, zipperfracs, and simulfracs.
The method assumes that microearthquake relative frequencies and magnitudes correlate to fracture evolution, but the methodology was adapted to account for microseismic events below moment magnitude (M) = 3, in which the log-linear earthquake frequency-magnitude relationship changes from slope (b) = 1 for naturally occurring quakes to b ranging from 1.5 to 1.8 for hydraulically induced microquakes (Equation 1). Hydraulic-fracturing activity level was determined by counting the number of cumulative seismic events above detection threshold for the stage with the worst detection sensitivity, ensuring that each stage is accurately compared.
Horizontal wells chosen for analysis were in a five-well pad in Ft. Worth basin. The wells were drilled into the upper Barnett shale to avoid the water-producing Ellenberger limestone. Wells were parallel and trended North-West. Separation between wells was about 500 ft. Wells A and B were simultaneously fractured, Wells D and E were zipper fractured, and Well C was singly conventionally fractured.
The wells were cased, cemented, and perforated at 50-ft intervals in 300-ft stages. The zipperfrac and simulfrac wells were fractured in 10 stages, while the single-fracture well was fractured in 11 stages. Pump rates were typically 50-60 bpm/stage with about 325,000 gal of water and 400,000 lb of sand per stage. Stages with values significantly lower than these were omitted from the analysis.
Completion of Wells A and B (simulfracs) occurred first, followed by the zipper fracs (D and E) then the single well (C). Well C, being in the center of completed wells, effectively represented an infill well. All stages were monitored seismically during fracturing for a total of 4,485 recorded events.
Results of the analysis showed that simulfrac wells underperformed the other completion techniques in terms of total number of seismic events (Fig. 4), indicating that these wells have smaller SRV. There was large variability in activity between stages (Fig. 5). The variability had no correlation to gamma ray (clay content), total injected water, or sand volume, suggesting that local stress factors govern much of the fracture stimulation. Quake counts increased with stage number, indicating that treatments improved during the stimulation process.
Stress evolution during fracturing stages was modeled by combining microseismicity, geomechanics, and flow analysis. The geomechanical model tracks stress in the reservoir during the treatment. Vertical stress (Sv), minimum horizontal stress (Shmin), and pore pressure (Pp) are determined from density logs, image and caliper logs of drilling induced tensile fractures and wellbore breakouts, minifracs, uniaxial compressive strength tests, and leak-off tests. Shmin was estimated from the instantaneous shut-in pressure (ISIP) of Well C measured at the toe of the well and confirmed by the ISIP from other stages.
ISIP increased during the treatments, from toe to heel, in a classic example of stress shadow behavior (Fig. 6). Simufrac wells had the greatest ISIP increase and zipperfracs had the least, with the single-fracture well generally in the middle.
Within wells, microseismicity and ISIP are positively correlated, but the trend does not hold between wells. The simulfrac wells had the highest ISIPs of the group but had lower overall quake counts at a given ISIP compared with the zipperfrac wells (Fig. 7).
One explanation for this result is in poroelastic changes during treatment. Large volumes of water pumped into wells during treatments may significantly control stress changes beyond simple planar fracture effects. For example, the simulfrac wells were fractured in half the time of the zipperfrac wells and thus only had half the time for fluid leak-off. Given the shorter leak-off time frame, simulfrac ISIPs are higher than the other frac techniques. Fig. 8 shows how quake count trends up with respect to hours elapsed since first stage completion, and this may explain how simulfracs time efficiency may work against stimulation effectiveness. Leak-off may be controllable through well selection, for example choosing wells separated by a large distance for simultaneous fracture stimulation to maximize microseismicity and therefore SRV. Alternatively, if well hits are a concern, adjacent simulfrac wells may be the preferable option to limit fracture growth. This is an area open for further research as simulfracs are further adopted in the field.
References
- Kim, A.S., Han., S., Ruhl, A., Belcourt, K., and Cazenave, R., “Simul-frac Journey in the Permian Basin,” URTeC 5340, Unconventional Resources Technology Conference, Houston, Tex., July 26-28, 2021.
- Russell, D., Stark, P., Owens, S., Navaiz, A., and Lockman, R., “Simultaneous Hydraulic Fracturing Improves Completion Efficiency and Lowers Costs Per Foot,” SPE-204138-MS, SPE Hydraulic Fracturing Technology Conference, virtual, May 4-6, 2021.
- Mandarich, M. and Neal, G., “Split-Fluid Method Improves Fracturing Operational Efficiency,” SPE Production and Operations Symposium, Oklahoma City, Okla., Mar. 27-29, 2011.
- Vermylen, J.P. and Zoback, M.D., “Hydraulic Fracturing, Microseismic Magnitudes, and Stress Evolution in the Barnett Shale, Texas, USA,” SPE 140507, SPE Hydraulic Fracturing Technology Conference and Exhibition, The Woodlands, Tex., Jan. 24-26, 2011.
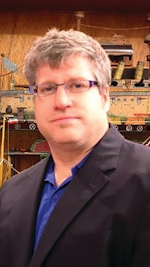
Alex Procyk | Upstream Editor
Alex Procyk is Upstream Editor at Oil & Gas Journal. He has also served as a principal technical professional at Halliburton and as a completion engineer at ConocoPhillips. He holds a BS in chemistry (1987) from Kent State University and a PhD in chemistry (1992) from Carnegie Mellon University. He is a member of the Society of Petroleum Engineers (SPE).