New SEG chief eyes mainstream role for full-waveform inversion
When asked about important geophysical trends, the new president of the Society of Exploration Geophysicists points to a seismic processing method called full-waveform inversion (FWI). But when asked to describe his involvement in FWI, Christopher L. Liner responds, "I'm an interested bystander."
The reason Liner, inaugural occupant of the Maurice F. Storm Endowed Chair in Petroleum Geology at the University of Arkansas, has no direct research role in FWI helps explain why the method has been conceptually available since the 1980s but only now eases into practical use.
FWI, a data-fitting technique that produces seismic-velocity images of the subsurface, requires a large suite of geophysical skills and enormous computing power.
"Maybe a dozen universities have the resources and computer power to do full-waveform inversion," Liner says. "Those kinds of integrated skill sets in the university setting are quite rare."
But as a geophysicist with experience in industry as well as academia, the SEG president sees practical value in a technique especially useful where rough topography confounds traditional processing of seismic data. Within 20 years, he believes, FWI will be a standard tool for developing velocity models.
Velocities and models
In traditional seismic processing, velocity models are not the primary goal; they're byproducts.
Normal processing seeks a representation of subsurface features, such as a visual image, detailed enough for interpretation to enhance decision-making about, for example, drilling targets and reservoir management. Those images normally result from the processing of seismic reflection data in steps performed sequentially in what Liner describes as a "decoupled processing flow." Velocity models, representing areas of different velocity within a specific earth volume, are side benefits of that work, useful in specialized operations but not basic tools of interpretation.
Seismic velocities are fundamentally important to geophysical work. They're the rates at which sound propagates through subsurface strata. Velocity data are essential to conversions of recorded data from reflection time to depth. And because velocities vary according to subsurface density and elasticity, they help characterize specific strata.
Near wellbores, velocities can be measured with procedures such as sonic logs, check-shot surveys, and vertical seismic profiles. Velocities for earth volumes between wells are derived mathematically during seismic-data processing.
But models based on velocities calculated during processing typically have low resolution; that is, they don't distinguish nearby features well. They therefore don't yield images useful for interpretation.
The higher-resolution images that are useful are essentially patterns of reflection points repositioned-or migrated-to correct spatially for the directional changes sonic energy makes as it propagates through layers of varying velocity. Usually, images resulting from processing and migration of reflection data serve interpretation well. Problems arise in land seismic where extreme surface and near-surface irregularity complicate statics corrections, which establish a stable elevation reference for migration and other processing steps. An early use of FWI has been to address those problems by accurately modeling near-surface velocities.
"When the situation is not so extreme, then the decoupled processing flow works," Liner says. "FWI is needed where the decoupled processing flow is inappropriate because the geology and topography are too complex."
The model as image
With FWI, the velocity model is not a byproduct of processing but rather the sought-after image.
"It's an end in itself," Liner says. "It shows you the velocities in depth so you can see the geology."
FWI achieves high resolution by "trying to match the full wave in its raw form," he explains. Unlike migration, which "tries to build an image from reflection events," the FWI image comes from "real velocities, real units….Those can be imported back to rock and fluid properties."
FWI builds a velocity model by mathematically modeling data using the best physics and computational methods available. The method next compares the model data with observed field data. It then repeatedly adjusts the earth-model velocities based on misfit of modeled and observed data. The converged product represents a velocity most likely to represent subsurface and, therefore, rock characteristics.
The computational load is high because the method mathematically tracks propagation of the full acoustic wave through a number of iterations. The cost, therefore, is high. But the product is an earth model with much higher resolution than is characteristic of normal velocity models.
The procedure, unlike more-routine processing, represents what Liner describes as "one grand, unified process."
Inputs for FWI are the observed seismic data, topography, and an initial velocity model. Calculations proceed directly from seismic data to the model, without usual processing steps such as normal moveout corrections, deconvolution, and migration.
FWI, Liner says, "gives you the best available near-surface model," he says. The method therefore maps drilling hazards well and "naturally gives you the best possible near-surface velocities" for use in traditional migration and other processing. "You can consider the velocities as a first iteration for the migration velocities," Liner says.
But commercial application of FWI remains limited.
"We're on the initial curve of full waveform inversion," the SEG president says. Although "it's well known in research departments," he says, "it hasn't really made it into mainstream exploration." In terms of progress toward widespread use by the exploration and production industry, FWI is "probably where 3D was 20 years ago."
Next steps
FWI usually is performed in 2D. Why not 3D?
"Everybody wants to do it," Liner says. "But it's computationally intense."
He says an FWI project in 3D is more costly than a 3D prestack depth migration, an iterative, computationally intense, and therefore expensive technique for creating images of especially difficult, steeply dipping subsurface features such as salt bodies.
A further challenge is not only to perform FWI in 3D but also to model the full elastic-not just acoustic-properties of the seismic wave.
"Theoretically, we know how to do elastic" modeling, Liner says. But the procedure involves the mathematical tracking of both the compressional energy, called P-waves, that dominates seismic work and less-commonly acquired data from transverse energy, or shear (S) waves. Acquisition of S-waves requires special, multicomponent receivers, which in marine surveys must be connected to the seabottom, a requirement that raises costs. And processing S-waves for FWI introduces another full set of calculations and iterations.
"It is a challenging computational thing," Liner says.
For FWI, though, the essential next step is 3D application of the method, for which only a few early examples now are available.
"Until you do 3D," Liner says, "you're just going to be a niche player."
Is moving FWI into 3D applications and, eventually, elastic modeling worth the cost? Liner answers pragmatically. "The question is how much additional benefit you get."
He adds: "We know how to do it now, but it needs to become mainstream." That, he says, will require more computer power and optimization of algorithms employed by the procedure.
Industry and academia
The new SEG president, a graduate of the University of Arkansas with an MSc from the University of Tulsa and a PhD from the Colorado School of Mines, began his professional career in 1989 as a geophysicist with Golden Geophysical, then Western Geophysical and Conoco. In 1990, he joined the faculty of the University of Tulsa, where he remained until joining Saudi Aramco as a research geophysicist in 2005.
He returned to academia in 2008 as a professor at the University of Houston and moved into his current position late in 2012. His research interests include carbonate outcrop and near-surface characterization; advanced seismic interpretation methods; seismic data analysis; and processing, anisotropy, and seismic-wave propagation.
Liner is the author of a book, Elements of 3D Seismology, and was the 1999-2001 editor of Geophysics. Besides SEG, he is a member of the American Association of Petroleum Geologists and American Geophysical Union and is an honorary member of the Geophysical Society and a corresponding member of the European Academy of Sciences.
Lean business
Asked to describe the status of geophysical work in the commercial oil and gas industry, Liner responds, "When you look at the big companies, the majors and second-tier companies, they're all over geophysics."
At present, he adds, geophysical contractors are being squeezed. "It's always been a lean business."
For small independent producers, "there's still room for improvement" in the acceptance and use of seismic methods." Because independent companies dominate unconventional resource plays, Liner adds, potential at times has been overlooked.
In unconventional plays, seismic methods can help identify not only sweet spots and fairways but also costly surprises such as unexpected faults. Unconventional resource development began with "spot drilling" and mainly "an engineering play," Liner says. "That's come around" for most of the industry.
For small companies, however, he adds, "we still need to get the message out there."
About the Author
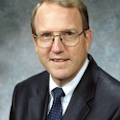
Bob Tippee
Editor
Bob Tippee has been chief editor of Oil & Gas Journal since January 1999 and a member of the Journal staff since October 1977. Before joining the magazine, he worked as a reporter at the Tulsa World and served for four years as an officer in the US Air Force. A native of St. Louis, he holds a degree in journalism from the University of Tulsa.